The European electricity mix is evolving fast. Once heavily carbon-intensive in the 1990s, the average electricity carbon intensity dropped to around 220 kg/MWh in 2023 [1]. Still, an ambitious target is set – to attain a carbon intensity of 100 kg/MWh by 2030 [2], see Figure 1. This rapid evolution has been a collective result driven by the deployment of new power plants using renewable energies to replace those using fossil fuels like coal and gas.
To succeed in the ongoing energy revolution and amid the absence of high-capacity storage solutions, we need to adapt our consumption to production over time. The FlexRICAN project is specifically focusing on the adaptation of Research Infrastructures (RIs) in Europe to this situation. Additionally, the project explores the potential of RIs, given their characteristic size, to deliver flexibility services.
To understand the concept of flexibility, which is one of the key pillars for effectively achieving the energy transition [5], we first should look at the Oxford definition of the word: “the ability to change to suit new conditions or situations.” Thus, we could consider the simple definition of energy flexibility given by Lund et al. [6] and NESO, who define energy flexibility as “the ability of an energy network to adapt its generation or demand in response to external signals.” This is further elaborated by Degefa et al. [7] as: “the ability of power system operation, power system assets, loads, energy storage assets and generators, to change or modify their routine operation for a limited duration, and responding to external service request signals, without inducing unplanned disruption.”. Thus, energy flexibility represents a low-cost (environmental and financial) approach to managing energy network imbalances that could potentially arise, given the proliferation of renewable energy systems. Additionally, it is key to point out that energy flexibility is not only relevant to grid operators but has implications for all stakeholders within the network, as shown in Figure 2 below.
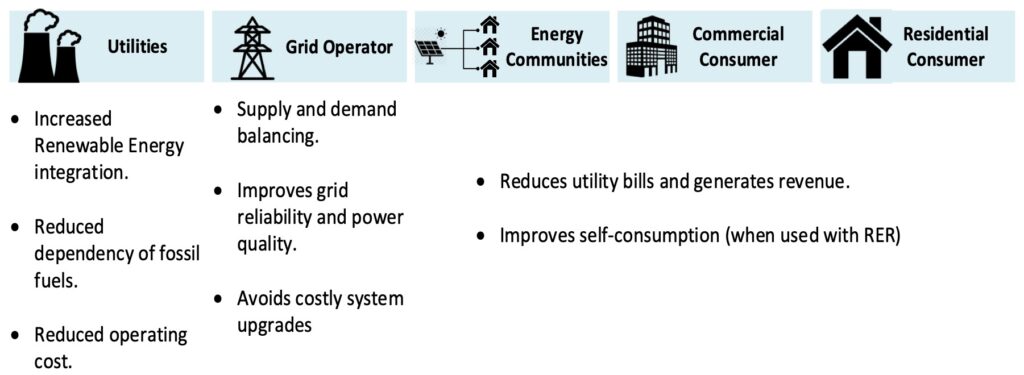
Although RIs cover a wide variety of structures, many of them can be described as electro-intensive facilities. In the FlexRICAN Consortium, their electricity consumption ranges from around 10 GWh per annum, as is the case of the 3 Extreme Light Infrastructure (ELI) facilities and the two continuous magnetic field facilities of the European Magnetic Field Laboratory (EMFL), to a forecasted annual consumption of 250 GWh of electricity per year in the case of the future European Spallation Source (ESS) in Lund, coordinating FlexRICAN project. The European Organization for Nuclear Research (CERN) remains a unique case with an annual electricity consumption of approximately 1300 GWh per annum.
In the FlexRICAN project, Research Infrastructures are not considered as dissociated points of consumption but as a network of energy nodes distributed across Europe. RIs play or can play a key role as energy nodes by contributing to energy generation, consumption, and storage, advancing sustainability and grid resilience. RIs can generate renewable energy through solar, wind, or experimental reactors, integrating clean energy into their operations and nearby grids. This practice is limited now, yet it offers great potential for the future.
As high-energy consumers, such as those with particle accelerators or supercomputers, RIs adopt energy-efficient systems to reduce their environmental impact – one example is the ESS. ESS aims to contribute to the district heating network by injecting the waste heat recovered from its operations, approximately 100 GWh of heat energy per annum, into the district heating network (see Figure 3). Otherwise, this amount of heat would have been released into the environment.
Additionally, the ESS intends to test cutting-edge energy storage solutions such as advanced battery supercapacitors and hydrogen energy storage systems. These energy storage systems will not only ensure the uninterrupted running of the facilities but will also facilitate the provision of grid ancillary services such as frequency and voltage regulation [9], [10]. Thus, by adopting sustainable practices and technologies coupled with spearheading innovation, RIs can be pivotal in enhancing the flexibility and resilience of the larger energy system (in this case, the European grid).
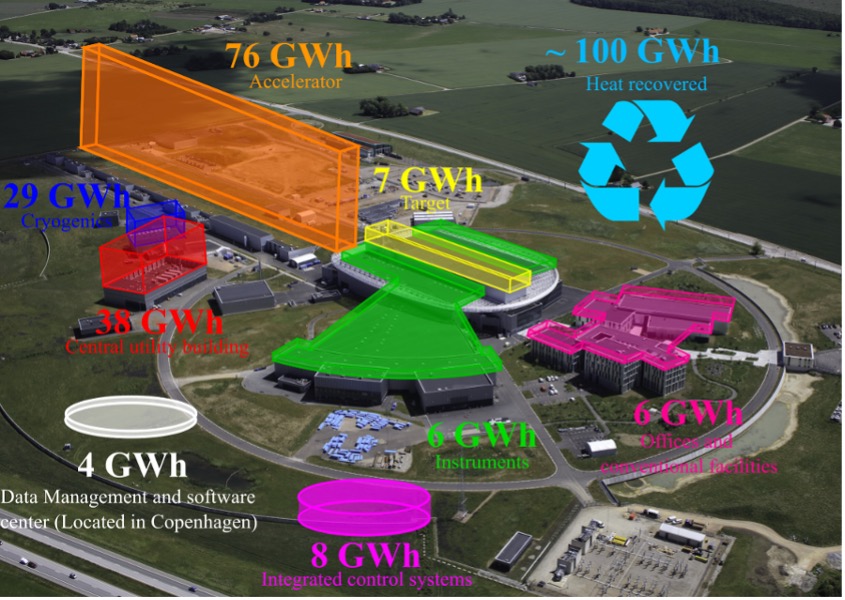
These research infrastructures are at the mesoscale between the European grid and local heat or electric energy networks. Based on this conceptual vision, the FlexRICAN project aims to develop open tools and methods for optimizing energy use (and effectively the carbon impact related to this energy use) within these infrastructures. One such tool is OMEGAlpes (Optimisation ModEls Generation As Linear Programs for Energy System), an open-source decision-aiding tool currently under development by the Grenoble Electrical Engineering Laboratory (G2Elab) [11].
The majority of RIs host users shaping the structure’s energy footprint. A stellar example is the Extreme Magnetic Field Laboratory (EMFL) facilities, where the use of continuous, intense magnetic fields is marked by high intermittency, as experiments operate on a “one user at a time” basis. As a result, the energy usage at the facility is directly linked to the types of research and the strategies adopted by researchers for their experiments. To elaborate on this point, two EMFL laboratories (located in Grenoble and Nijmegen) have a hyper-variable load profile and depend on the different researchers that use the facility. These experiments are planned months ahead and typically last for one to two weeks. Thus, there exists a potential to reduce the associated carbon impact from operating the high-field magnets by optimally planning the experiments at the facility. OMEGAlpes was used a posteriori to re-plan the experiments of the facility for the 2023 year and demonstrated that the carbon footprint of the facility could be reduced by approximately 22%. Although pedagogical, these results demonstrated that the facility could achieve a significant carbon footprint reduction through optimally planning its activities.
As a result, energy flexibility in RIs must take into account behavioural aspects and change management in addition to the different types of demands: the smooth running of the scientific experiments, the optimisation of the electricity purchase, integration of waste heat recovery or renewable projects, and participation in grid balancing mechanisms.
This diversity of external constraints implies that a purely mathematical optimisation of the RI energy consumption is insufficient to determine their optimal use of energy. A broader approach shall be developed that considers each RI as an energy community managed with specific and inclusive energy governance. Within the project, Deliverable 8.3 FlexRICAN Policy Brief will address this specific point by providing recommendations to the EU policymakers based on the project’s initial results and outcomes.
One of the strengths of the FlexRICAN consortium in developing an energy flexibility policy geared towards the stability of energy networks is that it brings together research infrastructures with very different consumption profiles:
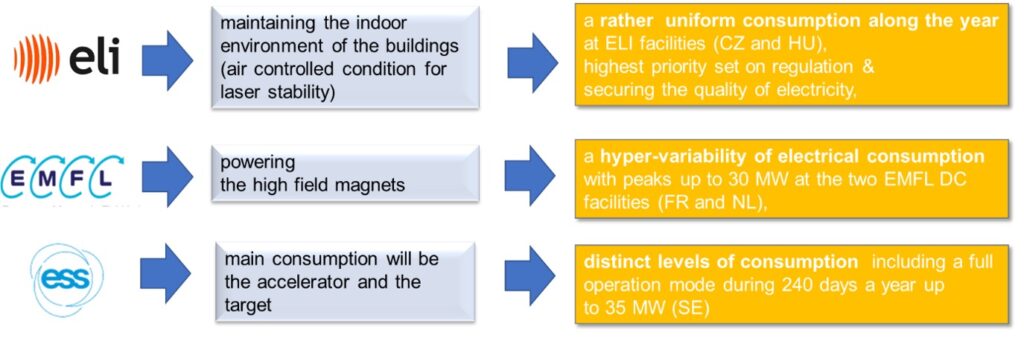
In addition, these research infrastructures are connected to regional power grids, and their specific features such as their energy mix can strongly influence the priorities for action as indicated in Figure 5.
It is worth highlighting the wide temporal range of energy flexibility mechanisms considered in the FlexRICAN project.
- Ultra-Short-Term (of the order of a second): the project is considering automatically controlled explicit mechanisms already used in industry but not currently in Research Infrastructures: frequency containment reserve (FCR), and automatic frequency restoration reserve (aFRR).
- Short-Term (Minutes to Hours): the planned energy storage solutions as well as the ability to halt temporarily some experiments as a voluntary consumption reduction measure can be considered.
- Medium-Term (Daily to Weekly): in this regard, Energy flexibility can then also be activated for electricity-heat conversion with characteristic times of the hour. Additionally, load-shifting strategies can be applied by rescheduling experiments within the same day (intra-day) as a means of demand-side response.
- Long-Term (Seasonal to Multi-Year): in this context, the project aims to optimally plan the use of facilities in order to take advantage of the seasonality that is prevalent in the energy mix and also associated with the various renewable energy resources (e.g. high solar production during summer).
The consortium includes two strategic industrial partners, Energy Pool, one of Europe’s leading companies in terms of demand-side energy flexibility aggregation, and a key player in the European energy flexibility market. The second partner, Alfa Laval, brings to the table their expertise in multi-vector energy optimization, especially in the area of heat transfer. These strategic industrial partners provide an additional layer of guarantee that all the different energy flexibility services available across the various RIs can be harnessed and applied to the relevant flexibility timescales during and after the FlexRICAN project.
Once ESS is fully operational, all three research facilities within the FlexRICAN consortium are expected to host approximately 5,000 users annually for research purposes. To achieve this, FlexRICAN aims to engage a broader stakeholder community, including users and staff from various RIs. The initiative plans to conduct training sessions as well as provide knowledge dissemination materials with a global aim of improving energy flexibility awareness in the research space. These knowledge dissemination exercises and tools are expected to lay the foundational groundwork for the establishment of mixed energy committees that bring together users, technical experts, and administrative staff from the facilities.
The FlexRICAN project highlights how European Research Infrastructures can drive energy flexibility and sustainability. By optimizing energy use, integrating renewables, and piloting innovations, FlexRICAN transforms RIs into active energy nodes that enhance grid stability and resilience via generation (using renewable energy sources or waste heat recovery), storage, and distribution.
Through collaboration, advanced tools, methods, and knowledge-sharing, the project sets a foundation for inclusive energy governance, positioning RIs as leaders and major participants in Europe’s green energy transition.
Authors
This article was written by François Debray, Dr. Eng. at the High Magnetic Field Laboratory in Grenoble (LNCMI). François is a Work Package Leader for Work Package 5: Waste Heat Recovery and Work Package 6: Energy Flexibility.
Contributions and revisions to the article were made by Frédéric Wurtz who is the Task Leader for Task 6.1: Direct Flexibility, Sacha Hodencq who is the Task Leader for Task 6.2: Indirect Flexibility, Severin Valla who is a participant for both Tasks 6.1 and 6.2 and Nana Kofi Twum-Duah who is a participant for the Task 6.1.
References for further reading:
[1] European Environment Agency, “Greenhouse gas emission intensity of electricity generation in Europe.” Accessed: Jan. 30, 2025. [Online]. Available: https://www.eea.europa.eu/en/analysis/indicators/greenhouse-gas-emission-intensity-of-1
[2] European Union technical Expert Group on Sustainable Finance, “Taxonomy: Final report of the Technical Expert Group on Sustainable Finance,” European Union, Technical Report, Mar. 2020. Accessed: Jan. 31, 2025. [Online]. Available: https://finance.ec.europa.eu/document/download/9101b639-ebe5-413e-9c86-3e291722899e_en?filename=200309-sustainable-finance-teg-final-report-taxonomy_en.pdf
[3] F. Ueckerdt, R. Brecha, and G. Luderer, “Analyzing major challenges of wind and solar variability in power systems,” Renewable Energy, vol. 81, pp. 1–10, Sep. 2015, doi: 10.1016/j.renene.2015.03.002.
[4] Md. S. Alam, F. S. Al-Ismail, A. Salem, and M. A. Abido, “High-Level Penetration of Renewable Energy Sources into Grid Utility: Challenges and Solutions,” IEEE Access, vol. 8, pp. 190277–190299, 2020, doi: 10.1109/ACCESS.2020.3031481.
[5] N. K. B. Twum-Duah, “Direct and indirect energy flexibility interactions at the building and community scale: From system to the human-interfaced system,” Université Grenoble Alpes, Grenoble, 2023.
[6] P. D. Lund, J. Lindgren, J. Mikkola, and J. Salpakari, “Review of energy system flexibility measures to enable high levels of variable renewable electricity,” Renewable and Sustainable Energy Reviews, vol. 45, pp. 785–807, May 2015, doi: 10.1016/J.RSER.2015.01.057.
[7] M. Z. Degefa, I. B. Sperstad, and H. Sæle, “Comprehensive classifications and characterizations of power system flexibility resources,” Electric Power Systems Research, vol. 194, p. 107022, May 2021, doi: 10.1016/j.epsr.2021.107022.
[8] “Background – Clean Energy Group.” Accessed: Oct. 14, 2022. [Online]. Available: https://www.cleanegroup.org/ceg-projects/energy-storage/background/#toggle-id-1
[9] Z. Li and F. Liu, “Frequency and voltage regulation control strategy of Wind Turbine based on supercapacitors under power grid fault,” Energy Reports, vol. 10, pp. 2612–2622, Nov. 2023, doi: 10.1016/j.egyr.2023.09.067.
[10] Y. Qin et al., “Lithium ion batteries participating in frequency regulation for power grid under the thermoelectric coupling degradation mechanisms,” eTransportation, vol. 19, p. 100290, Jan. 2024, doi: 10.1016/j.etran.2023.100290.
[11] S. Hodencq, M. Brugeron, J. Fitó, L. Morriet, B. Delinchant, and F. Wurtz, “OMEGAlpes, an open-source optimisation model generation tool to support energy stakeholders at district scale,” Energies, vol. 14, no. 18, 2021, doi: 10.3390/en14185928.
[12] Electricity Maps, “Electricity Maps – The leading resource for 24/7 CO2 grid data.” Accessed: Nov. 28, 2022. [Online]. Available: https://www.electricitymaps.com/